
One minute after the Big Bang, all the protons, neutrons, and electrons that would ever exist had been produced.
There were no atomic nuclei, though. No atoms. No materials as you and I know it–like wood, metal, or soil. Just a soup of subatomic particles and radiation.
The existing protons and neutrons could try to accrete together into an atomic nucleus, but they wouldn’t last long. High-energy photons–particles or “packets” of light–would quickly break them up.
But that was about to change…
As the universe continued to expand, it also continued to cool. And so did the photons it contained.
After the first 2 minutes of time, atomic nuclei could begin to form.
The first was deuterium, an isotope of hydrogen:

Hydrogen nuclei always have a single proton. But they can have different numbers of neutrons. “Isotope” is just a fancy term for nuclei of the same element that have different numbers of neutrons. Deuterium is an isotope with a single neutron.
Because the mass of subatomic particles is often described in atomic mass units (amu), where 1 amu is the mass of a proton, deuterium has a mass of 2 amu. We also refer to this as the atomic weight (even though, more generally, mass and weight are different things).
But deuterium is still just an isotope of hydrogen. Hydrogen was the only element that existed.
By the end of the third minute, though, deuterium was able to combine with other nuclei and form helium, the next element on the periodic table.
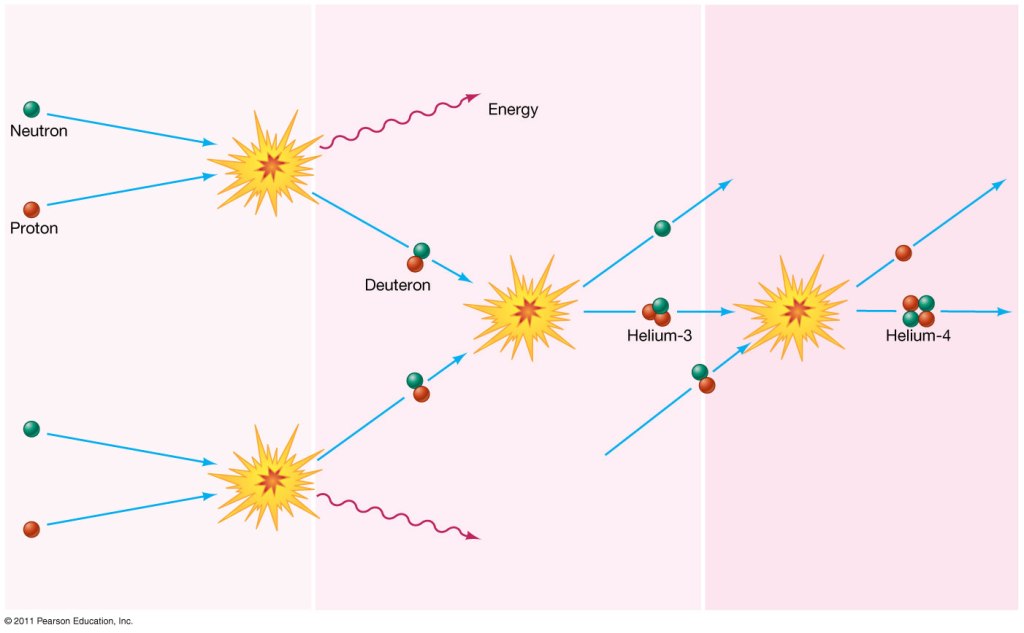
In the first step, a proton combines with a neutron, which releases energy and produces a deuterium nucleus.
Then the deuterium nucleus combines with a second deuterium nucleus. A neutron is ejected, leaving 2 protons and 1 neutron–a light helium nucleus. Because it is comprised of 3 particles, it is known as helium-3, and it has an atomic weight of 3.
Note that isotopes can also be shown as a superscript next to the element symbol, so helium-3 can be written as 3He.
Then, helium-3 combines with another deuterium nucleus. 2 protons and 1 neutron combine with 1 proton and 1 neutron. Add that together: in total, 3 protons and 2 neutrons combine. 1 proton is ejected, and the other 4 particles form a new nucleus.
This 4-particle nucleus is helium-4: a nucleus with 2 protons and 2 neutrons.
Note that helium-4 has an atomic weight of 4. And this is where we run into a problem.

This early element building had to go step-by-step, adding only one proton or neutron to the atomic nucleus at a time. And there are no stable nuclei of atomic weights 5 or 8.
Helium-4 was, largely, a dead-end.
Some nuclear reactions would have had enough energy to jump over step #5 and create a lithium-6 nucleus (6Li). Then lithium-7 (7Li) could be created. But it would have stopped there. The early universe couldn’t jump over step #8 and create a Berylium-9 (9Be) nucleus.
So, trace amounts of lithium-7 were created, but elements generally didn’t get heavier than helium-4.

But remember…it’s still been only 3 minutes since the beginning of time.
As the third minute passed, the universe continued to cool, enough so that nuclear reactions slowed down. And after 30 minutes, reactions had stopped completely. No more elements were produced.
Then how were the rest of the elements of the periodic table created, you ask?
Not in the early universe. They would have to wait millions of years for stars to form, when they would be cooked up in stars’ cores.
And that brings me to one of the critical pieces of evidence that gives cosmologists great confidence in our current understanding of the universe.
Theory and calculations predict that the early universe should have been roughly 75% hydrogen and 25% helium, with the tiniest trace amounts of lithium.
So, that is the atomic mixture that should have formed the first stars. But does theory match observation?

Using stellar spectra, we can study the composition of stars. And, indeed–the oldest stars observed are composed of roughly 75% hydrogen, 25% helium, and trace amounts of lithium.
Compare that to younger stars like our sun, which are composed of 73% hydrogen, 25% helium, and 2% heavier elements such as oxygen and carbon. These younger stars were part of a new generation of star formation, born from a mixture of elements that had been enriched by nucleosynthesis in the cores of the first stars.
In other words: theory exactly matches observation. And when theory matches observation, that gives scientists in general–not just astronomers–great confidence in their understanding of phenomena.
But the universe wasn’t done evolving. It would continue to expand and cool, leading into a new era–the interaction of nuclei and electrons, and formation of the first atoms.
We’ll get to that next week!