
When we talk about the first 30 minutes of time, we describe the universe expanding and cooling. As the universe cooled, the nuclear reactions that produced the first atomic nuclei slowed and stopped.
But it was only relatively cool.
The universe still held a temperature on the order of 1 billion kelvins–and that, by the way, converts to roughly 999,999,727°C, or 1,800,000,000ºF.
Ridiculous temperatures like those mean that the gases of the early universe must have been totally ionized. That is, electrons were not bound to atomic nuclei, and no atoms existed.
So how did we get to the universe we know today–a universe full of stars and galaxies?
The early universe was a soup of radiation and subatomic particles. And the density of radiation was greater than the density of matter.
Photons–that is, particles of radiation–constantly bounced off the free-floating electrons. This interaction meant their cooling was linked, and tied to the expansion of the universe. So matter couldn’t cool faster than the radiation. It also couldn’t clump together, because the radiation would smooth it out.
For 50,000 years, radiation dominated the universe.
But as the universe continued to expand, the radiation thinned out…and that’s when our old friend, dark matter, came into play.
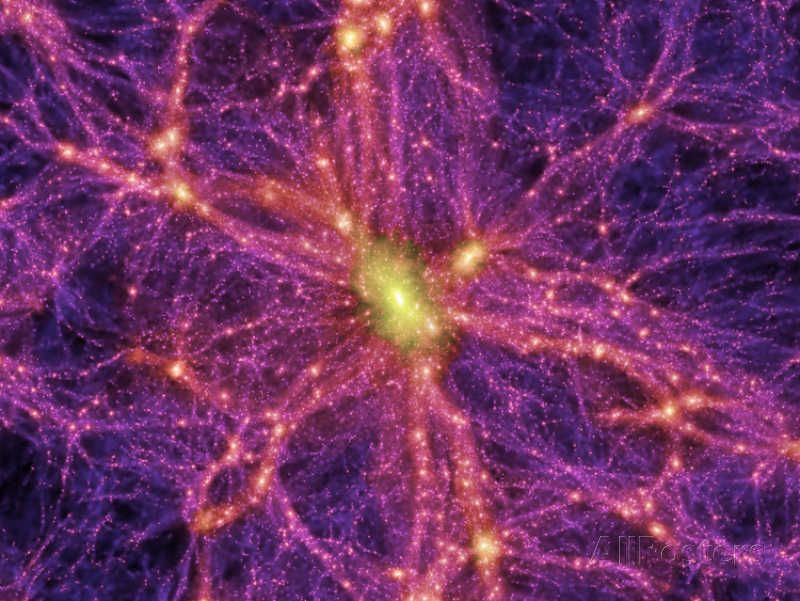
Remember way back in our exploration of galaxies, when I teased dark matter?
Yup. Told you we’d come back to it.
Dark matter doesn’t interact with radiation or with ordinary matter, so it was able to get a head start on clumping together. That’s what you see in the image above: dark matter clumping to form long strands, like a spider web.
And with radiation no longer spreading it thin, ordinary matter was drawn straight in like insect prey.
Under the gravitational influence of the dark matter web, ordinary matter began to form the clouds of gas that would eventually form into galaxies.
There were no galaxies yet, though. These gases were still ionized, and therefore opaque:

For another 35,000 years, the universe’s expansion carried the ionized gas apart–until the free electrons were too far apart to interact much with photons.
Now, photons could travel for thousands of parsecs without bouncing off an electron.
This is when the universe started to become transparent.
And at about the same time, the universe’s temperature fell to about 3000 K. For the first time, protons were able to capture and hold onto free electrons…forming neutral hydrogen.

And thus, the first atoms were formed.
This process is called recombination, but that’s not a very accurate term. It was the first time that protons were able to combine with electrons, so it should be called combination (no “re-” prefix).
As the protons snatched up all the free electrons, the gas was no longer ionized, and the photons no longer interacted with it. At this time, the universe became almost completely transparent.
Now, the gas continued to cool, but the photons retained the temperature of the gas at recombination: 3000 K.
Enter, stage left: the cosmic microwave background radiation.
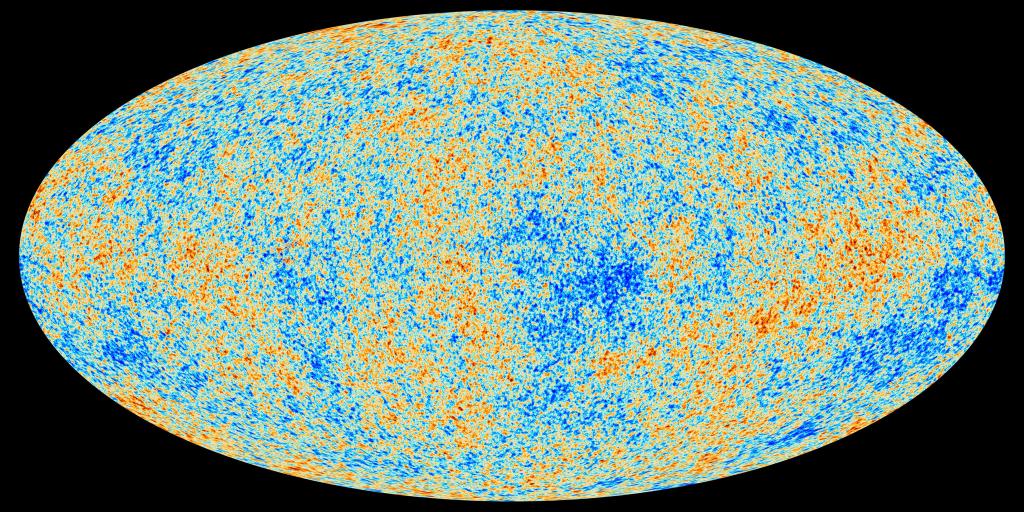
Remember a few weeks back, when we covered how the CMB was discovered?
This is what it’s from.
The CMB is the glow from recombination, when the first atoms formed. Those photons started their journey to Earth at a temperature of 3000 K. As they traveled, the expansion of space stretched their wavelengths into the microwave part of the electromagnetic spectrum. The CMB appears today to be about 2.7 K.
It’s also the farthest we can peer back through time, simply because before recombination, the universe wasn’t transparent enough.
We literally can’t see through the CMB. It’s like trying to look through a blackout curtain.

Next came an era we call the dark age.
The radiation from recombination retained its temperature as the CMB, but the gas continued to cool, all the way into infrared wavelengths. For about 400 million years, the universe expanded in darkness…
…until the first stars began to form.
This marks what we often call first light (or, as the graphic above puts it, cosmic dawn).

These first stars formed from a mixture of almost no “metals”–astronomy jargon for elements heavier than helium. Mathematical models predict that these stars would have been quite massive and bright, and they would have led very short, explosive lives.
In other words, light dawned on a universe in chaos.
Ultraviolet light from this burst of star formation would have ionized the gas–ushering in the era of re-ionization. And if we peer into the depths of the universe, beyond the most distant galaxies and even the most distant quasars, we can detect evidence of that ionized gas.
The re-ionization era marked the beginning of a new age: the age of stars and galaxies.

We’ve now followed the universe from its first one-millionth of a second, shortly after the Big Bang, to its 400 millionth year, when the age of galaxies began.
That’s a significant fraction of its current age. But it’s still just the beginning.
Next up, we’ll take a step back and explore what the universe looks like to us, today–and how our observations help us discover even more about its nature.