
Active galaxies are, without a doubt, absolutely spectacular.
Though rare, they tell a critical part of the story of galaxies–and of the universe as a whole. And we’ve spent the last few posts exploring them in depth.
But I know they can also be a bit perplexing. These are some of the most powerful and violent objects in the universe; it comes with the territory!
So, let’s boil this down to the basics and tie it all together. And let’s try not to get caught in a supermassive black hole in the process 😉
- What is an active galaxy?
- Types of active galaxies
- What triggers an eruption?
- How supermassive black holes erupt
- What we see
- The age of active galaxies
What is an active galaxy?
Something like this:
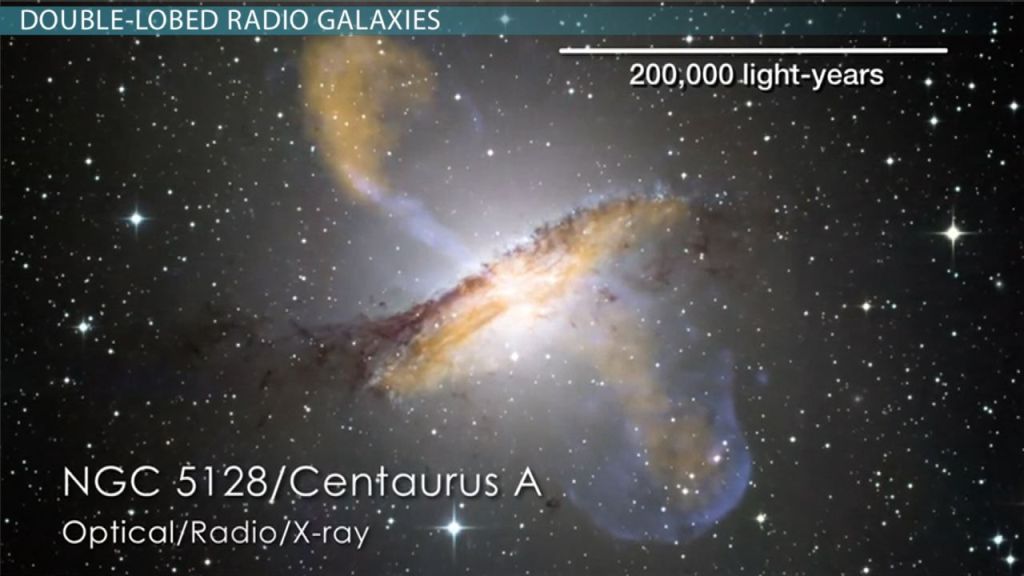
In a nutshell, an active galaxy is a galaxy whose nucleus is undergoing a powerful eruption.
Most often, when we refer to active galaxies, we refer specifically to active galactic nuclei (AGN). The nucleus is doing the erupting, not the outer parts of the galaxy. But even more specifically, the culprit is the supermassive black hole that lies at the heart of the galaxy’s nucleus.
Active galaxies usually look a bit “disturbed.” That is, they don’t look like one of the standard types of galaxies (spiral, elliptical, and irregular). Some appear twisted and distorted; others have strangely bright nuclei. Still others emit jets of gas traveling at relativistic speeds.
And some even look like stars–though they are not stars at all.
Types of active galaxies
There are several distinct types of active galaxies…
Seyfert galaxies
Seyfert galaxies are identified by their exceptionally bright nuclei. There are two types, distinguishable by their spectra.
Type 1

NGC 1566 is an example of a Type 1 Seyfert galaxy. This type of active galaxy makes up about 2% of all spiral galaxies.
They are bright in x-ray and ultraviolet wavelengths, but their most peculiar feature is in their spectra…

These galaxies have oddly broadened spectral lines.
Spectral lines are like fingerprints: we can use them to identify which elements of the periodic table an object is made up of. But they are usually sharp, narrow spikes, not these more mountainous spikes with broad bases.
These broad spectral lines are understood to be Doppler shifts, evidence that we are watching gas clouds orbit the galaxy’s center at exceptionally high speeds.
The brightness of these galaxies nuclei can also change very rapidly, indicating that they are extremely small. Exceptionally high-speed orbits in a very small volume of space is a telltale sign of a supermassive black hole.
Type 2
Type 2 Seyferts are pretty much the same as Type 1 Seyferts, except that their spectral lines aren’t quite as broad.
NGC 3081 is an example of a Type 2 Seyfert galaxy:

Astronomers think the discrepancy between the spectral line broadening of Type 1 Seyferts and Type 2 Seyferts is due to the angle at which we’re looking at the galaxy’s nucleus. If our view is obstructed by thick dust and gas, we might not see a strong Doppler shift from the innermost orbiting gas.
Double-lobed radio sources
Seyfert galaxies are primarily bright at their cores. But other active galaxies primarily emit energy from two external lobes…

…as in the case of Cygnus A, the brightest radio source in the constellation Cygnus.
In this case, the erupting supermassive black hole within Cygnus A’s nucleus is producing two oppositely-aimed jets of hot gas. These jets travel at a significant fraction of the speed of light.
The space around galaxies is full of extremely low-density gas called the intergalactic medium. Scattered throughout are pockets of even lower-density gas. Jets from active galaxies can plow open these pockets and impact the far side, creating two bright radio lobes.
Often, these jets and lobes are asymmetrical and twisted…
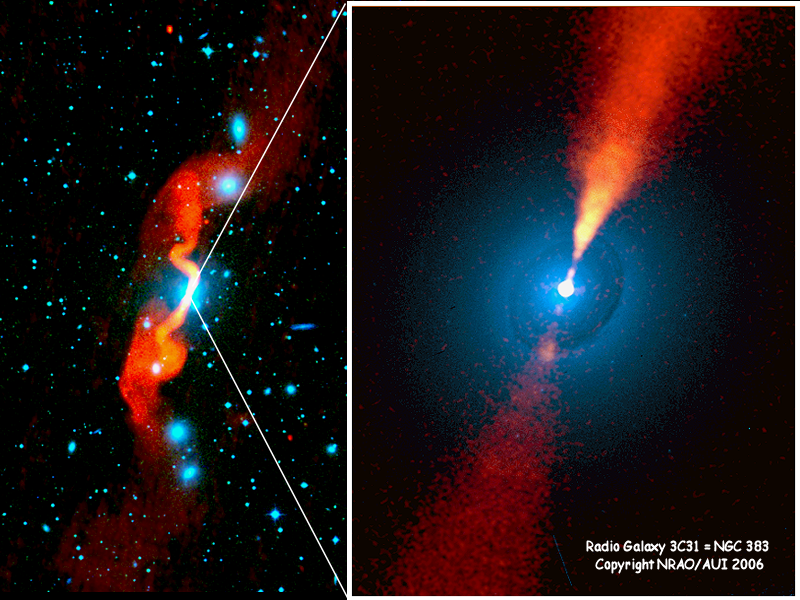
These patterns are understood to be due to motion of the active nucleus, likely because it’s orbiting some other massive object–like the nucleus of a nearby galaxy. The jets and radio lobes trail behind their source nucleus like streamers of ribbon.
Sometimes, we see two lobes but only one jet. This is likely because the jets are traveling at relativistic speeds and emitting photons in the direction of travel. If one is aimed straight at is, it will appear quite bright–but jet aimed away from us may be invisible to our instruments.
Quasars
And then, there are the most extreme of active galactic nuclei…quasars.
Quasar 3C 273 was the first object to be identified as a quasar:

Notice how it looks quite a bit like a star?
Problem is, it’s bright at radio wavelengths–which is very much not normal for a star. These objects also have a few strange emission lines in their spectra that posed quite a puzzle when they were first discovered.
They were dubbed “quasi-stellar objects,” a name that was soon shortened to “quasars.”
In 1963, Maarten Schmidt at Hale Observatories wondered if those strange emission lines were actually a familiar pattern, just redshifted due to the expanding universe. (We’ll get to the expanding universe very soon!)
So, Schmidt tried calculating redshifts for the Balmer series of spectral lines.
The emission lines from quasar 3C 273 clicked into place at a redshift of 0.158. And for the first time, astronomers began to unravel the mystery of quasars.

As it turns out, quasars are ridiculously far away–and to appear like stars from such distances, they must be some of the most luminous objects in the universe.
Not only are they about 10 to 1000 times the luminosity of our entire galaxy, they are smaller in size than our solar system.
Again, these are telltale signs of the presence of a supermassive black hole. But to be so bright, quasars must be undergoing an insanely powerful eruption.
Then…why do these supermassive black holes erupt in the first place?
What triggers an eruption?
Generally speaking, interaction between galaxies.



Remember how I said above that most active galaxies appear distorted? This is evidence of gravitational interactions between colliding galaxies.
But it doesn’t stop there…
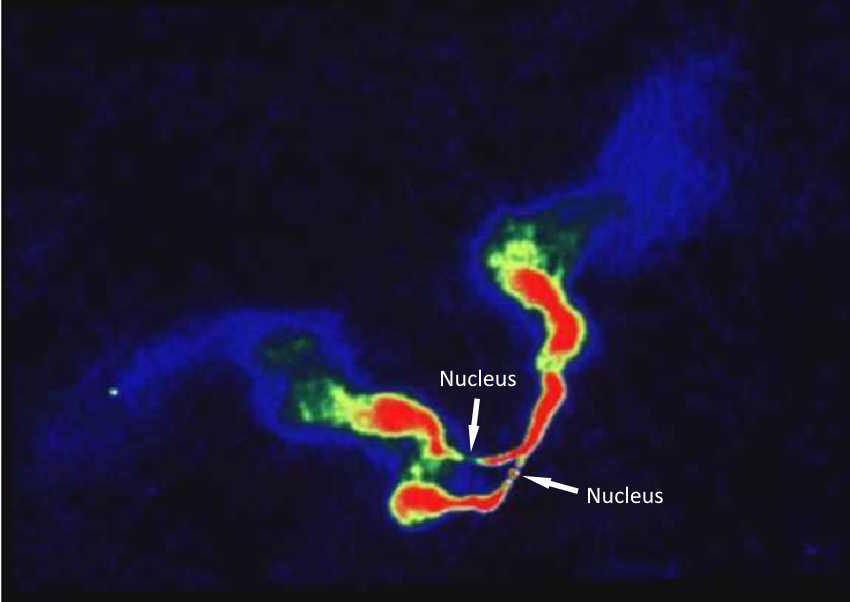
These two radio galaxies are in the midst of a merger. The actual galaxies aren’t shown here, only the nuclei within. Their nuclei are spiraling toward one another as their jets trail behind them like streamers.
ESO 146-5, too, is in the midst of a merger–between four separate galaxies.

And if you want to see what the resulting composite galaxy might look like, we need look no further than our old friend NGC 5128, the double-lobed radio galaxy.
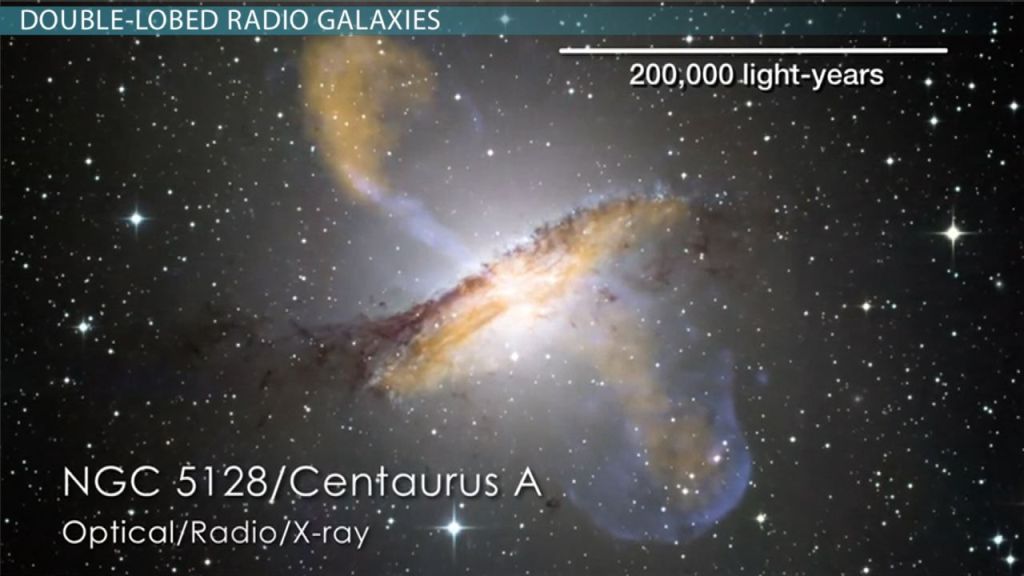
This active galaxy is the result of a merger between an elliptical galaxy and a spiral.
Most galaxies are home to a supermassive black hole. But the overwhelming majority of these black holes are “sleeping,” perfectly content to leave their host galaxies alone. They live off a starvation diet in the form of a meager, intermittent trickle of gas and dust.
But collisions and mergers between galaxies throw gas, dust, and stars inward, toward the sleeping supermassive black holes.
That wakes the dragon.
So, how do these eruptions work?
How supermassive black holes erupt
The first thing we need to understand about supermassive black holes is that they have extreme gravity.
The second thing we need to understand is that they work a bit like a bathtub drain: they are small, and so when material (for the bathtub drain, water) falls toward them, conservation of angular momentum forces it to swirl into a whirlpool.
Conservation of angular momentum
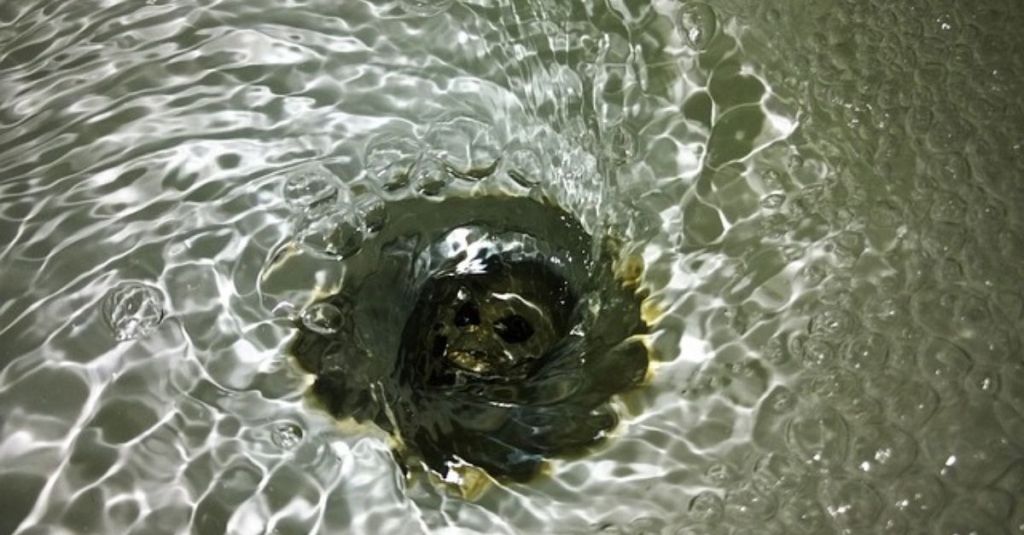
For a black hole, this means that material from its host galaxy–gas and dust–forms an accretion disk around it.

Such an accretion disk would form around any normal, stellar-mass black hole. But supermassive black holes have millions to billions of times the mass of our sun.
That insanely strong gravity forces infalling matter to spin a heck of a lot faster than it would around a stellar-mass black hole. As the particles of the accretion disk pick up speed and collide with other infalling particles, the temperature of the accretion disk skyrockets.
And there’s a general rule in astronomy that if gas is superheated like that, it must be at least partially ionized. That is, electrons have been stripped from their atomic nuclei.
Anatomy of the accretion disk

In the region closest to the black hole, there is no possible stable orbit, and any orbiting particle must ultimately spiral into the black hole. So, immediately surrounding the black hole is an empty cavity.
Beyond that is the innermost region of the accretion disk itself. This region is where it’s hottest–where the central black hole’s gravity is the strongest and wreaks the most havoc.
When matter is hot, it tends to expand, because the particles have high kinetic energy and bounce off each other, effectively pushing each other away.

Models indicate that the innermost part of the accretion disk should be no different. This region should puff up a bit like a donut.
Beyond that innermost region is a “sweet spot”: conservation of angular momentum forces the accretion disk to flatten, but temperatures aren’t high enough to counteract that. The disk stays flat. Which means, of course, that this intermediate region of the disk is also cooler than the center.
And then, we have the outermost part of the accretion disk: a fat, cool donut shape much more pronounced than the inner region, made up of dusty gas.
But here’s the million-dollar question.
How the heck do we get from accretion disk to active galactic nucleus?
Eruption
It all comes down to the ionized accretion disk. An ionized gas can trap magnetic fields–just like the way the sun’s rotation twists up its magnetic field to produce the sunspot cycle.
Research into how supermassive black holes work is still in its infancy, but it seems that magnetic fields get trapped in the ionized accretion disk and are drawn inward.

The result is powerful magnetic tubes extending along the disk’s axis of rotation. These tubes confine and focus jets of hot gas and radiation.
And that’s how we get an active galactic nucleus.
What we see
I’ve searched online for explanations of how we get the different types of active galaxies, and there doesn’t seem to be much of a unified consensus–yet. But it does seem that, for the most part, the kind of active galaxy we see depends on our viewing angle of the supermassive black hole.
That doesn’t necessarily mean the viewing angle of the galaxy. The black hole and its host galaxy could have different axes of rotation and be tipped at an angle to one another.

Different sources I’ve found disagree on which viewing angle produces which types of galaxies. But one thing is certain: in order to see a double-lobed radio galaxy, we need to see the accretion disk edge-on.

That way, the jets and radio lobes will appear to either side of it–not in front of and behind it from our vantage point. If one jet is faint or invisible, then it must be angled somewhat away from us, but we should generally be seeing the accretion disk edge-on.
It’s also understood that we see Seyfert galaxies when we can just barely peek over the edge of the accretion disk, into the black hole’s maw.
As for quasars, my textbook describes them as the product of us staring “down the dragon’s throat,” directly into a relativistic jet.
But where do active galaxies fit into the story of galactic evolution?
The age of active galaxies

Remember what triggers a supermassive black hole to erupt: material is flung toward it and forms an accretion disk.
And remember how material gets flung toward it?
When the universe was young–within billions of years after the Big Bang–it had not expanded much. The infant galaxies of the time were much closer together, and much more likely to collide.
This was the “age of quasars.”
These were the collisions that produced today’s large, “mature” galaxies, so the active galaxies of the time were small. Even then, powerful eruptions would’ve been rare.
Though they are not common, some quasars are found at look-back times when galaxies had barely begun to form. The universe was small, but galaxies were rare enough that collisions were unlikely. These earliest quasars may be evidence of the initial formation of the first supermassive black holes, before galaxies had been built around them.
But quasars are not seen at small look-back times, in the present-day universe. There’s no way to get rid of a supermassive black hole…so where did they all go?
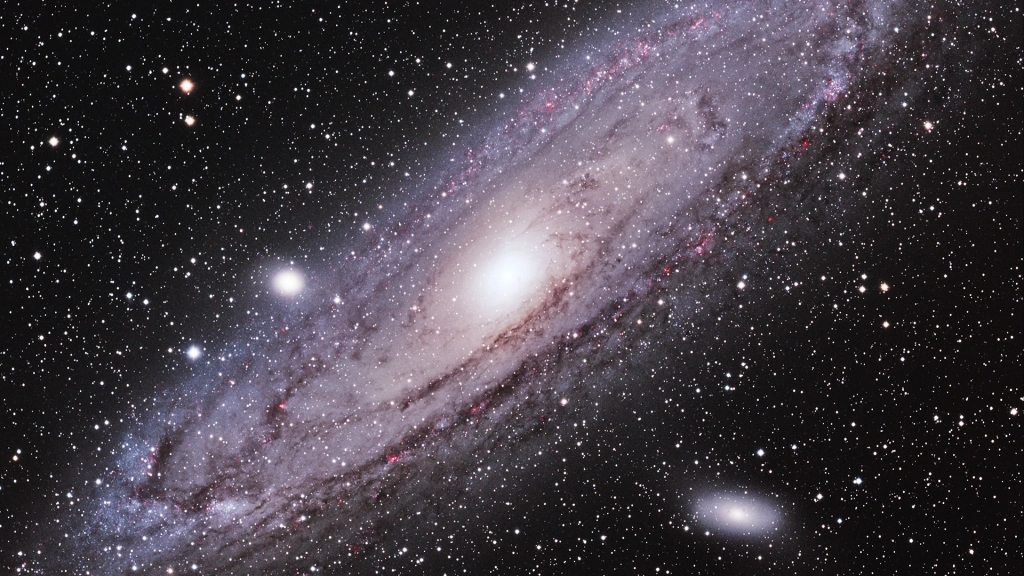
They are all around us, deep in the hearts of the trillions of “ordinary” galaxies. Our own Milky Way and our neighbor Andromeda may have once been home to a quasar. The dragon has simply gone to sleep.
Active galaxies are evidence of a short-lived stage of galactic evolution that any galaxy can undergo.
And there we have it, people–the full story of galaxies and their evolution. Now we’re finally ready to dive into cosmology…next week!
. . . glad there’s not a quiz . . .
LikeLiked by 1 person
I hope it wasn’t too confusing? (There’s no stakes here, only your own enjoyment of learning. I wouldn’t include a test of any sort.)
LikeLiked by 1 person
No, not too confusing.
Just attempting humor. I was never a class clown in my youth, so I’m trying to make up for it in my old age.
LikeLiked by 1 person
Haha, no worries. I getcha. I just wanted to make sure, since the primary goal of this blog and especially “demystified” posts is to clarify things! 🙂
LikeLike